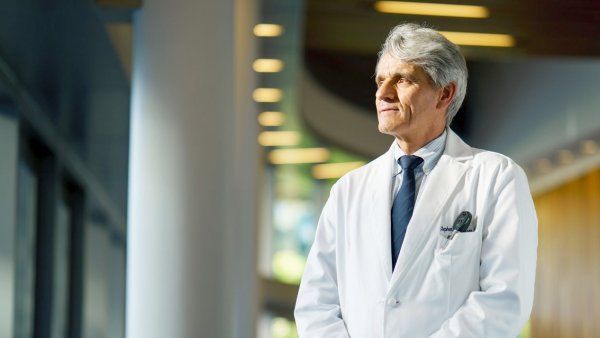
Innovation can be born of necessity, conscience, creativity, luck, or more likely, all of the above, all at once. Whatever the impetus, the active ingredient of invention is collaboration.
The five scientists highlighted here – bioengineers Tejal Desai and Shuvo Roy, MD/PhD candidate Mozziyar Etemadi, microbiologist Joe DeRisi, and physician/surgeon Michael Harrison – trace intersecting paths, tapping each other’s expertise nearly constantly.
When technology and need collide, Harrison sees alliances with engineers as a portal to a new realm of efficacy. “When we have a problem and there’s a missing piece, we don’t just wonder if someone will come up with it someday,” says Harrison. “We just go out and do it, together.”
Detecting Early Labor
Preterm labor is the number-one cause of infant mortality worldwide, taking the lives of 13 million babies a year. More shocking still is that the chances of having a preterm baby in the United States – one in eight – are greater than the global risk of one in 10.
These are the statistics that drive the BirthAlert team, which consists of a perinatologist, a mechanical engineer, an electrical engineer, and bioengineers. They’re rethinking the way we detect labor.
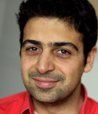
“We’ve been predicting labor by the same measures – dilation and contractions – since the dawn of time,” says Mozziyar Etemadi, an MD/PhD student in Shuvo Roy’s bioengineering lab. “Once they set in, you only have 24 to 72 hours before the baby comes, and that can be too little time to intervene.”
This is especially true for women in the developing world who may be a long way from a hospital, according to Larry Rand, MD, leader of the BirthAlert team and director of UCSF’s Perinatal Services and the Fetal Treatment Program. “In my work in Liberia, all too often I encountered women who came in contracting in the throes of preterm labor and were therefore stuck in their village,” says Rand. “The only way out is often a 13-mile bike ride, which a woman in labor can’t do.”
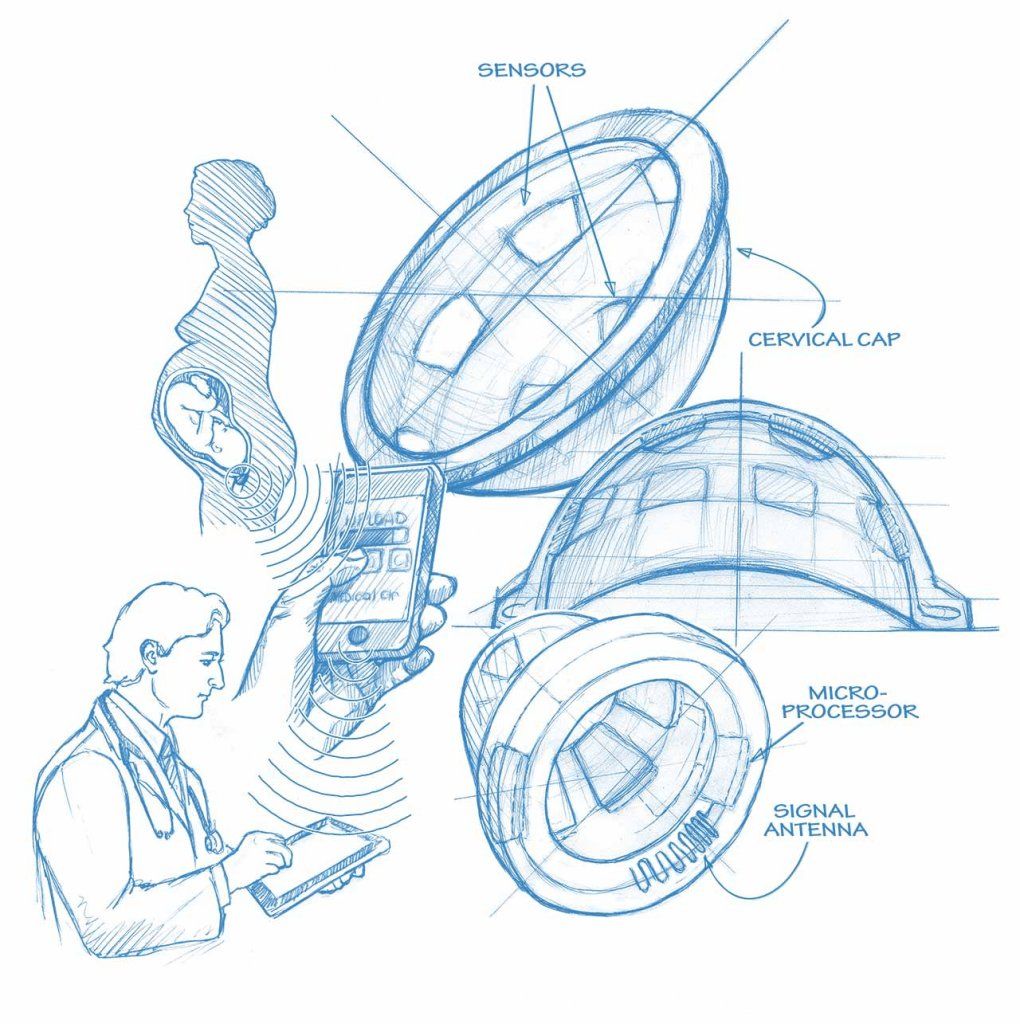
The BirthAlert team has come up with a smart cervical cap that will monitor an earlier sign of impending labor – changes in the collagen of the cervix. Collagen, which is in many of our tissues, can be hard, like it is in our nose, or soft, as it is in our ears.
“During the course of a pregnancy, collagen in the cervix changes from rigid to soft, then the cervix opens. So the collagen essentially goes from gate to gateway for the baby,” says Etemadi.
Rigged with sensors, a small battery, and some wireless equipment to get a signal out to a phone, the device can be inserted briefly at home, once a day, without the aid of a professional. If softening is detected, the device will transmit a signal to a cell phone, which will then send the information to the physician.
“The normal process of softening to opening takes anywhere from one to three weeks,” says Etemadi, giving the physician a far wider window to work within.
Just exactly how much BirthAlert has widened the intervention window in high-risk pregnancies will likely be shown in the device’s first clinical trials, which started in spring 2012 after receiving ethical approval by the UCSF Committee on Human Research. It’s being tested in women deemed at risk of preterm birth, including those with previous preterm pregnancies and women over the age of 35 – all of whom will have BirthAlert inserted briefly for a reading during their regular prenatal visits.
If proven effective, BirthAlert may not only save millions of lives, but could also give better warning signals for the babies who do make it yet face a life of disability because of early birth, according to Rand. In addition, the device would reduce the formidable costs associated with preterm birth – an estimated $26 billion a year in the United States alone. Estimated cost of the device: $20 to $30.
Ending Dialysis
Be it financial, humanitarian, or ethical, the case for Shuvo Roy’s artificial kidney is clear. Not only would the implantable, coffee cup–sized device eliminate the need for dialysis – which costs Medicare $30 billion annually – but, as Roy notes, “It’s impossible not to be moved by the plight of the patients.”
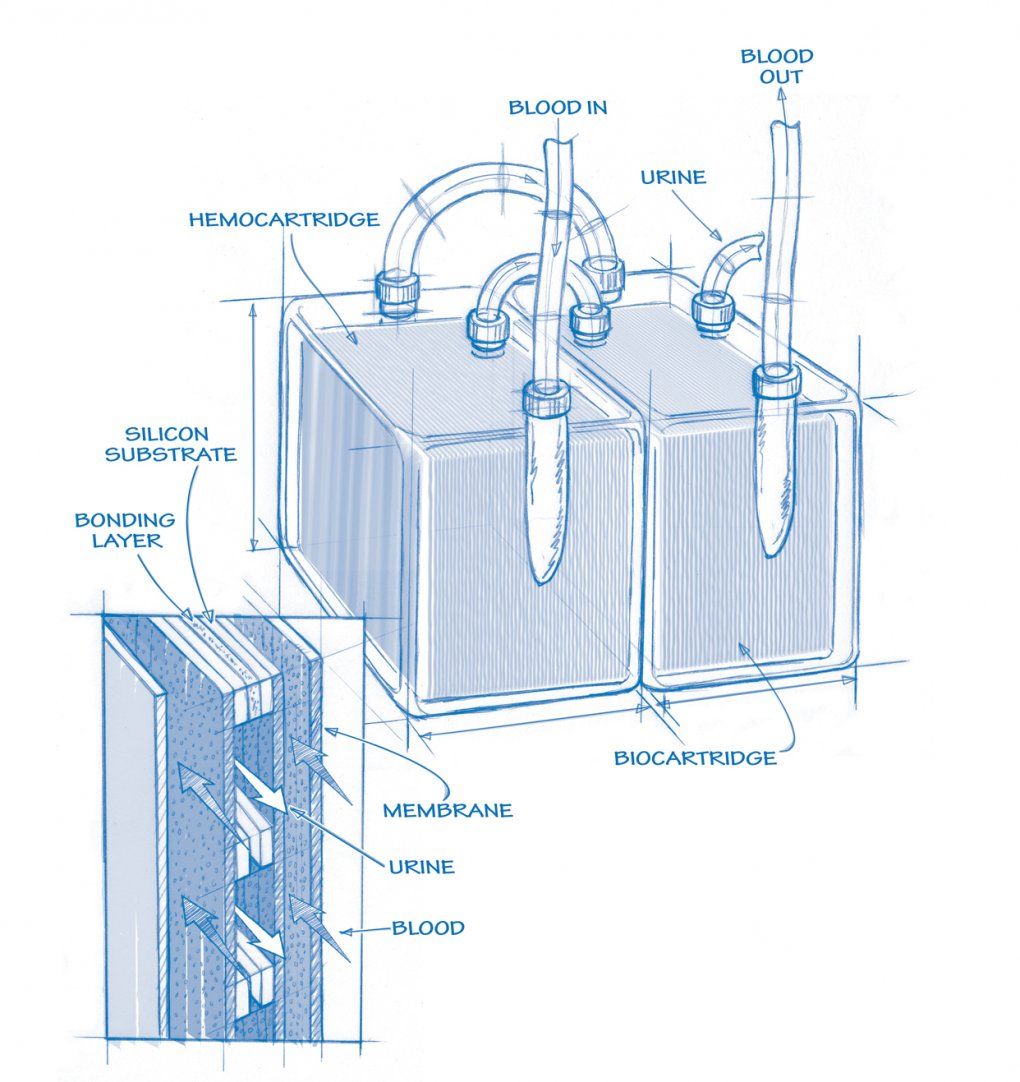
Artificial Kidney (See larger)
Dialysis patients, the sickest of those diagnosed with end-stage renal disease (ESRD), can be hooked up to the machine as many as three days a week, three to five hours a session. “The annual mortality rate is around 20 percent, close to that of colon cancer,” says Roy, PhD, associate professor in the Department of Bioengineering and Therapeutic Sciences, a joint department of the schools of pharmacy and medicine.
Dialysis patients are in desperate need of a rare commodity – kidney transplants. The statistics are devastating: 17,000 organs are available; 92,000 patients are in line. With ESRD rates increasing at 5 percent to 7 percent a year, the odds grow worse by the day, and the costs are enormous. This might explain why Roy’s kidney project qualified for the Food and Drug Administration’s Pathway Program, which essentially fast-tracks the approval process.
The device under development looks so simple – like an oversize nine-volt battery – yet a decade’s worth of dramatic bioengineering advances have shaped it. Surgically implanted into the abdomen to establish a permanent blood connection, it provides continuous therapy without immunosuppressant drugs.
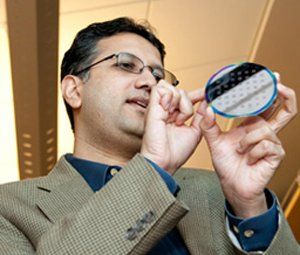
The first major component of the device is the hemofilter, which uses silicon nanotechnology to produce a membrane that blocks toxins without causing clotting. The filter works at a pressure driven by the patient’s heart, eliminating the need for a power supply. The other major component, the bioreactor, mimics the metabolic and water-balancing duties of the kidney.
Building a successful bioreactor required a reliable supply of human-derived cells. “Silicon membranes had to catch up with the needs of the project. And we had to understand how to isolate the kidney cells and store them outside the body,” says Roy. “Those two developments combined with advances in materials for biocompatibility – the right coatings – are what make the device viable now.”
Tejal Desai’s early work on the biocompatibility of silicon was, according to Roy, seminal in the field of miniature implants in general and, more specifically, critical to the long-term success of the device.
So far the proof-of-concept components of the artificial kidney have worked successfully in small animals, and extended studies are planned for the immediate future. With a green light on these studies and adequate funding, Roy expects human trials to begin in five to six years.
Directing Drug Delivery
Picture this: as you approach your front door, it looks oddly crooked. On the other side stands your spouse, whose face seems wavy and distorted. These are among the unnerving first signs of age-related macular degeneration (AMD), the leading cause of blindness in people over 60. Although new drugs can stop AMD’s progression, physicians have struggled with getting them to the right place inside the body.
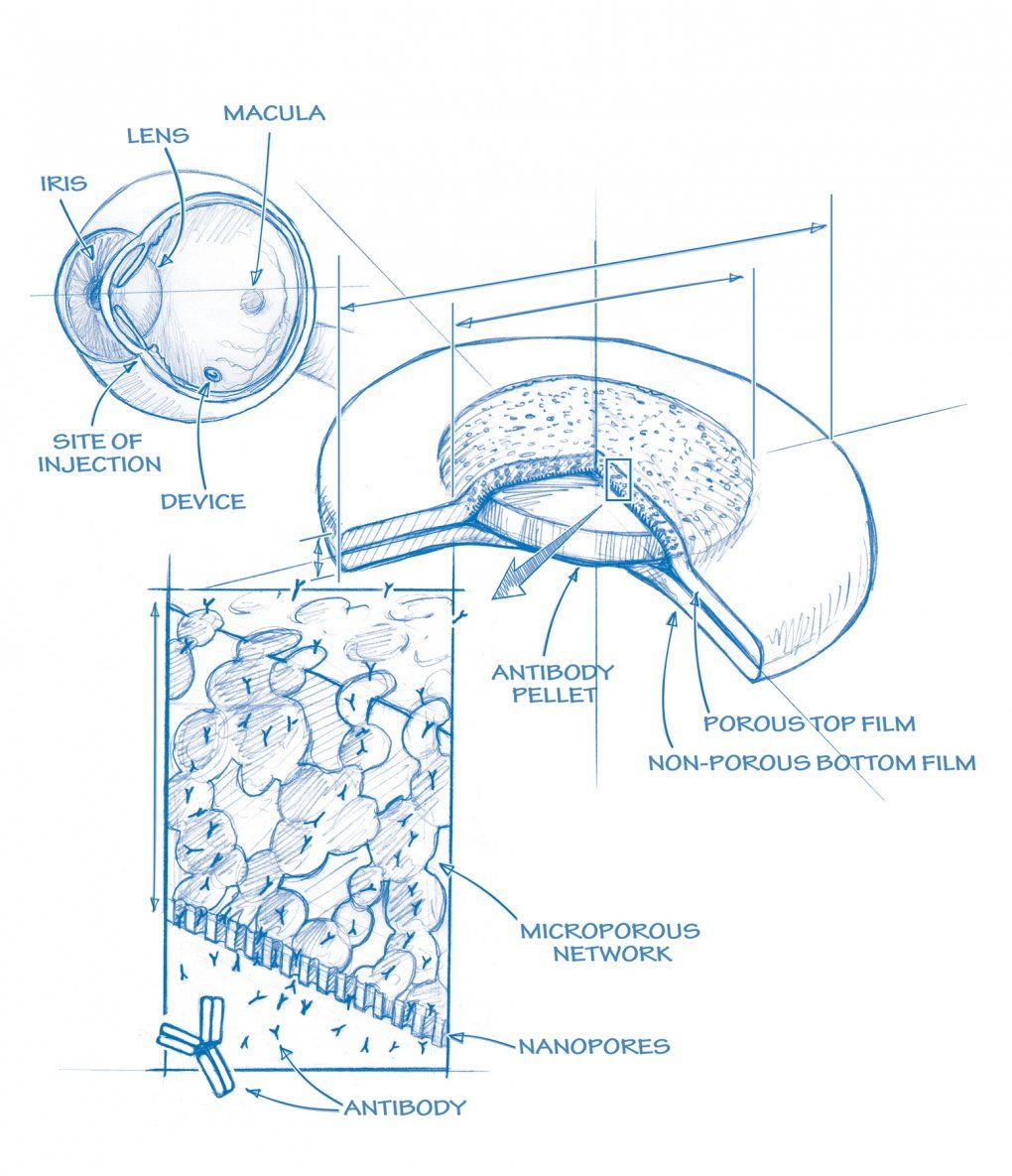
Drug Delivery Device (See larger)
Tejal Desai has a tiny solution. “I attended a forum shortly after I came to UCSF, where several ophthalmologists talked about challenges in their field,” recalls Desai, PhD, professor in the Department of Bioengineering and Therapeutic Sciences, a joint department of the schools of pharmacy and medicine, and co-director of the Master’s in Translational Medicine program. When it was the engineers’ turn to discuss what they do, she described her work: using nanofabrication technology to create a microscopic drug delivery device, chemically engineered to release interferon over several months, as a treatment for hepatitis patients. “An ophthalmologist came up to me at the wine and cheese gathering afterwards and said, ‘If you could do that within the eye, it would be a major revolution.’”
That was three years ago. Now Desai has partnered with the ophthalmologist who approached her – Robert Bhisitkul, MD, PhD – to create a prototype and file a patent for a drug delivery device for macular degeneration. Currently, the only way to get a drug to the retina is to inject it directly into the area.
“Because injected drugs actually break down and clear away quickly, patients have to have the injection monthly,” says Desai. “We are developing a polymer thin film that is injected in the back of the eye and can deliver drugs for at least six months at a time.” The film eventually biodegrades, and patients return for another injection.
The device has the potential to make a substantial impact. “One, you’re able to use less drug, so there will be major savings in pharmaceutical costs in the health care system,” explains Desai. “Two, with a more direct delivery, you get a more effective response. And three, you get fewer side effects because you’re using less drug.”
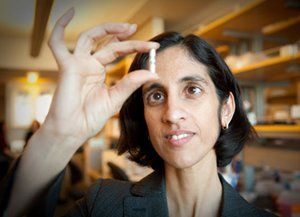
The device is currently being tested in animal models; human trials are expected in three to five years.
Desai’s next frontier – diabetes. Since very little (less than 5 percent) of a drug taken orally actually makes it into the body, these patients are forced to endure daily insulin injections. “The insulin would be broken down in the intestine before hitting its target site,” she says. Desai is now working on an artificial pancreas housing cells that can sense and respond to changes in blood glucose, doing away with insulin injections for a lifetime.
Easing Breath
Necessity has been the mother of all of Michael Harrison’s inventions. As co-founder of the UCSF Fetal Treatment Center, Harrison, MD, has racked up a list of devices that changed children’s lives before they took their first breaths.
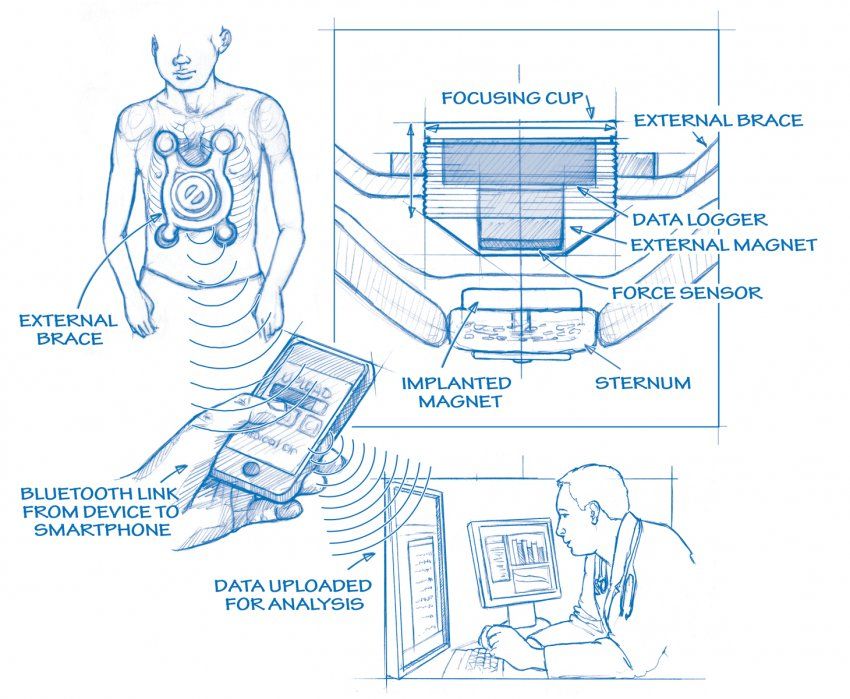
Magnetic Minimover (See larger)
“The whole field of fetal surgery was built around the innovation of devices,” says Harrison. Take the early surgical stapler: tests showed that using metal staples to close a uterus caused infertility in animals. “It was a shocking revelation, so I worked with a company on the East Coast for a year to solve the problem,” he recounts. “They came up with a stapling device that became the enabling agent for fetal surgery. That device is what made fetal surgery real.”
Likewise, need forced the invention of tools for the minimally invasive procedures of fetoscopic surgery, where surgeons operate with elongated tools and scopes, following their movements on a screen. “The instruments – traditional scissors and graspers but on long sticks – were part of the explosive era of innovation between 1980 and 1990,” says Harrison. “They’re used not just for fetal surgery but for any arthroscopic procedure.”
Harrison is still at it, inventing devices for an underserved population – children. His latest, the Magnetic MiniMover, is innovation born of conscience. It’s designed for kids who suffer from a condition called sunken chest, which can compress and damage the lungs and heart.
“All we have to fix sunken chest is a terrible operation, where we rip a child’s whole chest wall apart and put it back together with a steel bar,” says Harrison. “I knew we had to work with a different principle.”
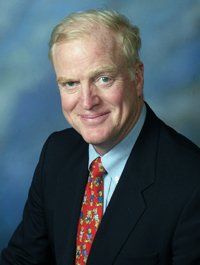
His solution, the Magnetic MiniMover, works by perpetual force, much like braces work to correct a child’s crooked teeth. In an outpatient procedure, Harrison surgically implants a magnet in a child’s sternum. He then gives the child an opposing magnet to wear at night, or even during the day under clothing, to slowly but surely draw the chest wall outward. Harrison worked with Mozziyar Etemadi and Shuvo Roy to rig the device with sensors that send messages about usage and pressure to the child’s physician, via cloud database technology. The device is now in clinical trials.
As is the case with most pediatric devices, the impact appears small on the surface: only 2,000 children a year are diagnosed with the disease. “This is a good example of why it’s so challenging to develop a pediatric device,” explains Harrison.
Yet the US Congress considers the need to be great – leading it to mandate the creation of a Pediatric Device Consortia Grant Program to stimulate the development of these devices. Harrison directs the UCSF branch of the consortium. “No big company is going to invest, because there’s no pot of gold at the end of the rainbow. But there will be a safe treatment that’s a whole lot better than the old one.” And that’s more than good enough for Harrison.
Isolating Viruses
The global impact of Joe DeRisi’s ViroChip is measured in what hasn’t happened. The device holds DNA samples of every known virus on the planet (there are 22,000). Within minutes, the ViroChip can detect any virus – like severe acute respiratory syndrome (SARS), which DeRisi worked with the US Centers for Disease Control and Prevention to identify in 2003 – leading to its isolation and containment, and potentially preventing a pandemic.
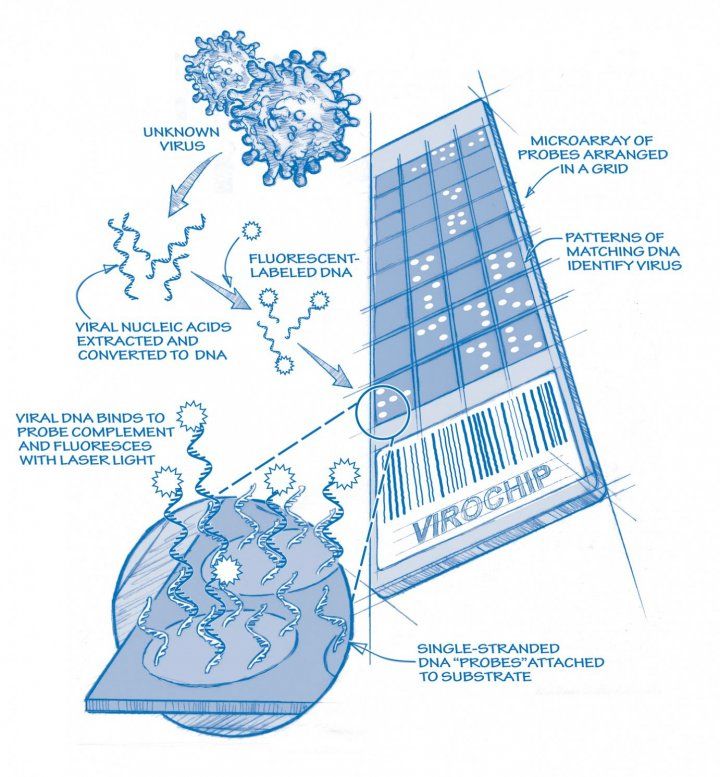
Virochip (See larger)
Since SARS, several new viruses have been identified by the ViroChip, including some associated with diseases like gastroenteritis and severe respiratory disease – two of the biggest killers of children under 5 years of age.
“The ViroChip has called into question the role viruses may play in cancer and other diseases,” says DeRisi, PhD, co-chair of the Department of Biochemistry and Biophysics and a Howard Hughes Medical Institute investigator at UCSF. “Are they causal, or do they just coexist? We can’t say yet, but it’s a question worth asking.”
Looking like little more than a microscope slide, the ViroChip relies on DNA to make match-ups. When the DNA in a patient culture, which is labeled with a fluorescent, sticks to its corresponding virus DNA on the slide, matches are made – and many a mystery has already been solved.
DeRisi, who not only built the ViroChip but the robots that assembled it, is now working on software technology that will push the library of bioinformatics currently available to sequence vast quantities of biologic material, mining for viruses.
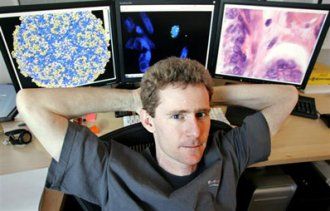
“The idea is, here are some sick people, and the sickness could be contained in one area of the body – say the gut,” says DeRisi. “In this case, we’re sequencing everything that’s there, looking for the needle in the haystack – the virus.”
DeRisi believes the technology will be a lens to a whole new viral frontier. “With the ViroChip, we can find viruses that are close enough to known viruses to be recognized,” he explains. “In our new system, we have pulled up viruses that look so different, we weren’t sure they were even viruses. We’re building software that’s able to put the pieces together and confirm they are.”