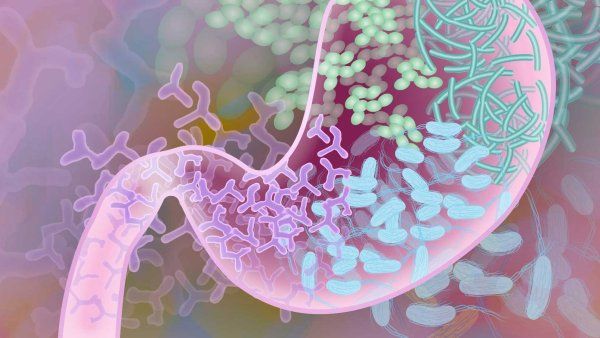
Long ago, when Andrew Goldberg, MD, was a resident, the ear, nose and throat specialist had a patient who came in repeatedly with a chronic infection in one ear. The man had been prescribed all manner of treatments – from vinegar drops to antibiotics to antifungals to steroids – none of which provided lasting relief. Then one morning, the patient walked into the office and asked Goldberg to take a look in his ear – the infection was wiped out. “Don’t you want to know what I did?” Goldberg recalls the patient asking, with a grin.
“So he tells me,” Goldberg continues, “I took some wax from my healthy ear and stuck it in my bad ear. Within a few days, my problem was gone and never came back.’ Of course I laughed it off, thinking that the infection had spontaneously cleared and that the guy was crazy,” reflects Goldberg, who is now the director of Rhinology and Sinus Surgery at UC San Francisco.
Decades later, when he began investigating the myriad bacterial communities thriving in the human body, Goldberg realized what a clever, if not desperate, move his patient had made. His good ear hosted an abundant and stable microbial community, while his bad ear had a depleted population of microbes that left it in a chronic inflammatory state. The bacteria in the wax from his good ear had brought the other ear back to healthy harmony.
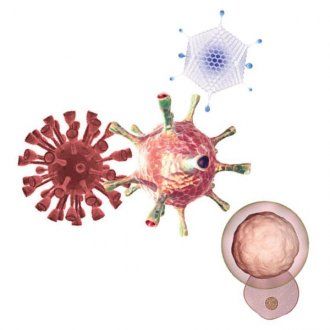
That patient’s recovery hints at the enormous therapeutic potential of the human microbiome – the 100 trillion bacterial cells living in and on our bodies. Such cells outnumber the body’s own cells 10:1. They are housed primarily in our gut, where roughly 70 percent of the components of our immune system reside. Scientists are hard at work trying to leverage the extraordinary healing powers of the microbiome, mining it for treatments of a variety of conditions, including asthma, irritable bowel syndrome and obesity.
And for good reason, according to microbiologist Michael Fischbach, PhD. “One-third of all human medicines are made by bacteria,” he says. “Clearly, they are the best chemists on the planet.” His lab studies how simple microorganisms create drugs with such proficiency. “Over the past 20 years, people have done seminal work uncovering which genes enabled microorganisms to synthesize wildly complex drugs,” he says. “For me, the trick is to be able to find other genes that look similar enough that I know they are there to make a drug.” The process used to be an arduous one, involving a great deal of luck while combing for bacteria through the soil or the ocean, where the vast majority of such drugs have traditionally been found.
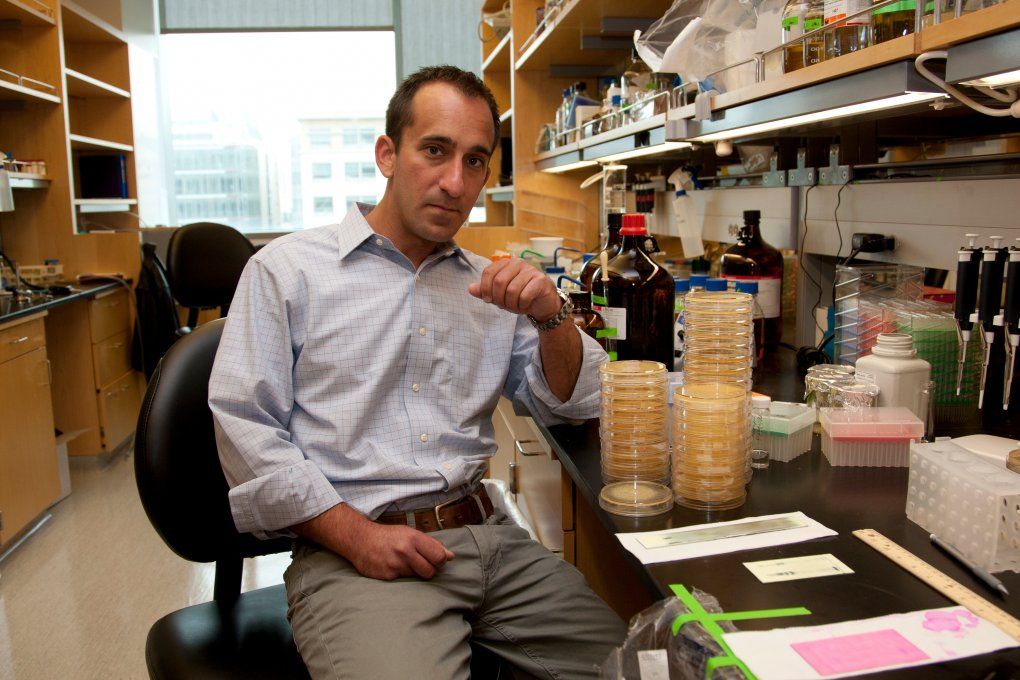
Michael Fischbach, PhD. Photo: Cindy Chew
That all changed with improvements in genetic sequencing and computational technology. Now, Fischbach uses his computer to scan every bacterium whose genome has been sequenced for drug-producing genes. As expected, his searches have turned up many drug-producing genes in ground- and marine-dwelling bacteria.
“But I was shocked to see so many in the human microbiota. You used to have to travel to the coast of Palau to mine the ocean sediment for drugs,” says Fischbach, an assistant professor of bioengineering and therapeutic sciences. “Now we can just check our gut!”
Embracing Our Inner Pharmacy
Day in and day out, gastrointestinal microbes go about the care and maintenance of our bodies by extracting vitamins from the food we eat, goading our immune systems into action when need be, and producing the anti-inflammatories and antibiotics that keep us well. “The human microbiome is basically a drug factory,” says Fischbach. He is hard at work trying to discover its best products.
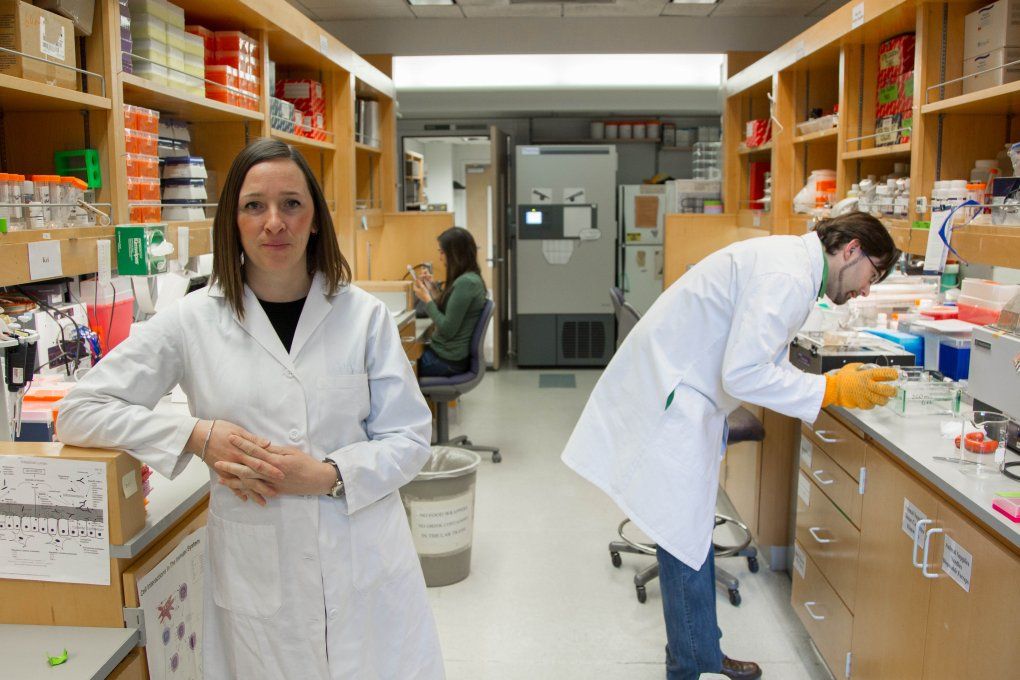
Susan Lynch, PhD, and her team study the microbial populations that inhabit the human body. Photo: Susan Merrell
He and UCSF Executive Vice Chancellor and Provost Jeffrey Bluestone, PhD, an immunologist, study a very promising gut bacterium called Bacteroides fragilis. It produces a chemical that resembles a well-known immunomodulatory drug that was discovered in a sponge off the coast of Japan 20 years ago. Fischbach and Bluestone, who holds the A.W. and Mary Margaret Clausen Distinguished Professorship in Metabolism and Endocrinology, predicted that the chemical would have a similar effect on the immune system, and they were right: The chemical produced by B. fragilis interacts with a type of human immune cell called a natural killer T cell. That meant it could be very useful in treating allergic and autoimmune diseases.
Lactobacillus johnsonii is another gut bacterium with extraordinary therapeutic potential. Susan Lynch, PhD, an associate professor of gastroenterology, homed in on the organism when trying to explain a curious fact: Children who grow up in homes with dogs are less likely to develop allergies and asthma. She designed a study that involved exposing two sets of mice to household allergens – one set to dust from homes with dogs, and the other set to dust from homes without dogs. “We found that mice exposed to the dog-associated house dust were protected against allergic inflammation or infection with a virus associated with the development of asthma,” says Lynch, who directs UCSF’s Colitis and Crohn’s Disease Microbiome Research Core.
To figure out what microbes may have been at work, Lynch compared the bacterial fingerprints of the two sets of mice and found that approximately 100 different organisms were present in higher levels in the gut microbiomes of the protected mice; one of them was Lactobacillus johnsonii. She isolated this species from the mouse gut, fed it alone to another set of mice, and found that this resulted in airway protection, though to a lesser extent than exposure to dog dust did.
“This tells us a number of important things,” says Lynch. “First, that the Lactobacillus johnsonii did not act alone – it requires specific microbial partners to afford full airway protection. It also suggests that this full suite of protective organisms in the human body are not all native; they presumably can be sourced from the environment.” In other words, Lactobacillus johnsonii partners with bacteria from the dog-household dust to fight off pulmonary allergens. According to Lynch, this makes perfect sense, given that a bacterium often relies on other bacteria to produce certain compounds, such as the amino acids it needs to survive.
Mob Rule
Overwhelming evidence suggests that one of the hallmarks of chronic inflammatory disease is an overall depletion of microbes. That’s why introducing a single organism into a microbial ecosystem that is out of whack may not always be effective; its partners may have been wiped out by the illness or, paradoxically, by an antibiotic intended to cure it.
“We are after the core set of microbes that are essential to creating a healthy microbial ecosystem,” says Lynch. “Our data supports the idea that Lactobacillus represents an anchor or keystone organism in such communities.” Its presence, in other words, dictates which other organisms are present and how they behave – and that ultimately influences a person’s immune response.
In fact, Lactobacillus johnsonii is known to play a powerful role in newborns’ immune systems even before their first breath. “Lactobacillus johnsonii represents one of the major species in the microbial inoculum that a vaginally born newborn receives,” says Lynch. “We think it’s critical in structuring or priming development of the microbial community in early life, the period during which the immune response matures.” Proof of its importance can be measured by its absence. Children born by cesarean section – and therefore not exposed to Lactobacillus johnsonii or other potentially protective species in the mother’s vaginal tract – have a 30 percent higher risk of developing allergic asthma during childhood.
Bacteria by Prescription
Clearly, Lactobacillus johnsonii has a long and proven history of having a positive therapeutic effect on the body. But what might a microbial agent look like if it were crafted specifically as an asthma drug?
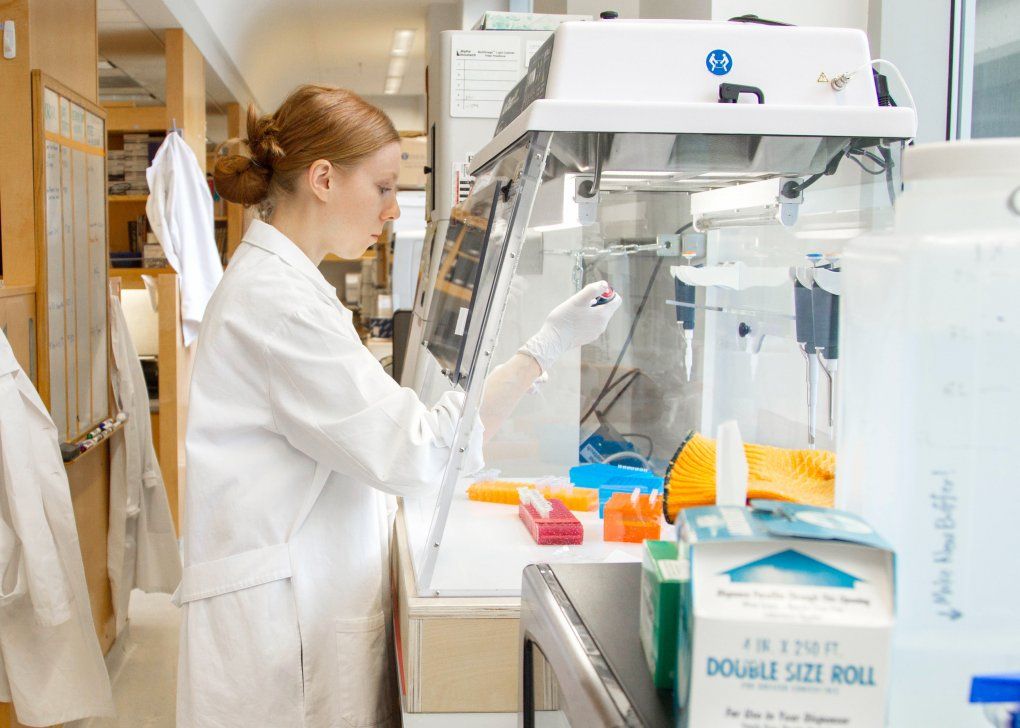
Kaitlyn Lucey, PhD, a postdoc in the Lynch lab, works to generate a fluorescent strain of the bacteria Lactobacillus so its presence can be tracked in the mouse gut. Photo: Susan Merrell
The first consideration would be when to intervene. Though diseases such as childhood allergic asthma do not manifest clinically until a child is about 6 years old, Lynch believes that their genesis lies in events that occur very early in life. “Essentially, we are trying to build and sustain an appropriate microbial bioreactor in the infant gut,” says Lynch. “We would likely begin in early life, from birth, with supplementation strategies that manipulate the microbial community in a way that benefits the child.” Such a therapeutic might take the form of a dehydrated but viable microbial species mix, not unlike current probiotic supplements, which would be added daily to the infant’s diet. It would be given from birth through the first few months of life, since that seems to be the critical period in the assembly of a hardy, healthy microbial community.
Even after the right microbes are introduced, they would have to be maintained to give them staying power. “We also need to investigate nutritional interventions to promote the longevity of these organisms that we are introducing into the system,” explains Lynch. “These are known as prebiotics – the complex carbohydrates and fibers that fermentative organisms, like the Lactobacilli, prefer to gnaw on.”
There are prebiotics on store shelves right now, but, like the probiotics next to them, they are fairly blunt tools. The prebiotics of the future will be specifically tailored to the nutritional preferences of the bacteria that are being used as therapeutic agents. “Right now, prebiotics are typically broad-spectrum complex carbs that increase the presence of certain beneficial organisms that readily metabolize them,” says Lynch. “But this will only occur if those organisms are present in the first place; many good bacteria are depleted with disease and inflammation.”
Best Bet
The treatment from the human microbiome that’s closest to being realized is for a condition caused by a bacterium called Clostridium difficile. It’s a rare and painful affliction of the bowels that typically occurs as a result of antibiotic treatment. “Antibiotic use depletes the gut of some of the important components of its community and allows for overgrowth of C. difficile, a pathogenic bacterium,” explains Andrew Goldberg. This causes gastrointestinal distress, diarrhea, and fluid loss – and can be fatal.
You used to have to travel to the coast of Palau to mine the ocean sediment for drugs. Now we can just check our gut!”
The current protocol calls for still more antibiotics. However, recent research done in the Netherlands may ultimately change that. In a well-controlled trial of patients with C. difficile, half were given antibiotics and the other half received fecal transplants from a healthy individual, typically someone in the same household who had been exposed to the same environmental bacteria. “The study was stopped before completion,” says Goldberg, “because the effectiveness of the fecal transplant was so high that it was felt to be unethical to continue the standard treatment in those patients.” Physicians believe that this finding will also have very positive implications for patients with other gastrointestinal diseases, such as inflammatory bowel disease. There are currently several ongoing trials of fecal transplantation in the United States. If the efficacy of the treatment proves to be as good as or better than the current protocol for patients with recurrent C. difficile, the FDA would likely fast-track the fecal transplant, says Lynch, making it immediately available.
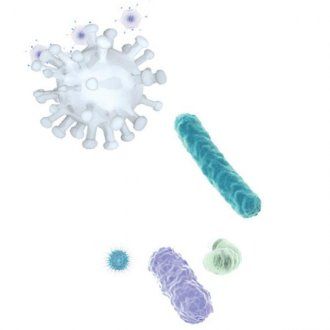
Until other treatments derived from the human microbiome are honed into viable therapeutics, antibiotics will likely remain the treatment of choice for many of the illnesses that plague us. At first glance, the two strategies appear to approach such illnesses from opposite directions: Antibiotics kill the bad actors, while probiotics and prebiotics bolster defenses to restore order. And some argue that antibiotics take out the good with the bad, potentially pushing a microbial community further off kilter, as is the case with C. difficile. “We found in mice that antibiotics actually made it easier for a pathogen to grab hold and to create a disease state,” says Goldberg, primarily because the good actors hadn’t survived to intervene.
Yet Fischbach predicts that, ultimately, the two approaches will be combined. In the future, he believes, antibiotics will likely be followed by what he calls a “probiotic-chaser,” to help re-assemble the microbial community, while prebiotics may be used for health maintenance.
In the meantime, the field needs to figure out exactly how microbes engage our immune system to make us well, according to Fischbach. Only then will we be able to make effective use of a resource that has been, quite literally, under our noses as long as we have walked the planet.
“Drug companies spend an enormous amount of time and money trying to develop a single drug and get it into your gut at an appreciable concentration,” observes Fischbach. “Yet we have bacteria already in our gut, probably producing dozens of drugs and affecting our biology and health in profound, very specific ways. Understanding how that works, I hope, is the legacy of what we are doing in this field right now.”