
Cancer sucks. Chances are you know someone who’s had it, or it might have threatened your own life. Too often, a diagnosis demands answers to impossible questions. Which body parts are you willing to live without? Would you risk feeling awful for a while, presuming the treatment might buy you more years of life? Would you settle for one year? How about a few months?
The same cellular feats that can help your skin stitch itself back together are also at work in tumors. The challenge is to kill the cancer cells – which are the patient’s cells, just dividing wildly – without also killing the patient. Unfortunately, cancer can be as complex as we are. Worried about, say, one genetic mutation making your cells go rogue? Try many mutations, all helping the cancer thrive at your expense. That’s not unusual. And there are hundreds of types. People don’t get “cancer” anymore. They get, say, triple-negative breast cancer characterized by the absence of estrogen-receptor, progesterone-receptor, and human-epidermal-growth-factor-receptor-2 expression.
Scientists no longer expect to find a convenient, one-size-fits-all cure. But they are finding ways to outsmart this disease. Whether in five years or 50, advances in research underway now could transform how humans cope with cancer.
Click a button or scroll down to explore these breakthroughs.
Implants and Pumps
Keeping Toxic Treatments Where They Belong
The side effects of traditional cancer treatments can be serious. Hair loss. Weight loss, sometimes courtesy of regular vomiting. Loss of red blood cells, also known as anemia, which causes exhaustion. Some treatments for cancer are so toxic that they make you toxic; writer Caitlin Flanagan described her first round of chemotherapy as an “absolute hell” that included instructions to avoid hugging her children.
Chemotherapy and radiation work by stopping cancer cells from replicating so rapidly. Unfortunately, some other, rather important cells – like your hair follicles or the lining of your digestive tract or your bone marrow – also divide quickly. That’s why researchers are working to corral cancer treatments and the damage they can do.
For example, cancers in the lining of the abdomen can be difficult to treat with typical chemotherapy, which circulates throughout the body. The latest approach, offered by UC San Francisco’s Mohamed Adam, MD, goes like this: Surgeons take out obvious tumors, pump chemotherapy right into the abdomen, then drain it back out. The idea is to bring the battle right to the cancer’s doorstep but leave surrounding neighborhoods unscathed.
Surgeons are also implanting pumps during operations for cancer that’s spread to the liver, offering patients a way to get chemo exactly where they need it – at doses much higher than they’d get with systemic treatment. Ajay Maker, MD, head of UCSF’s Division of Surgical Oncology and the Maurice Galante Distinguished Professor, hopes to test newer immunotherapies with pumps, too.
Another potential breakthrough: tiny, silicone-based implants that deliver steady doses of a drug. Clinical trials for the implants are underway for prostate cancer patients at the National Cancer Institute, with plans to develop a similar initiative for breast cancer.
Prostate and breast tumors are often treated with drugs that disrupt sex hormones. These drugs can also prevent breast cancer in women with BRCA mutations, who face an exceptionally high risk of developing the disease. Unfortunately, like other systemic treatments, the drugs don’t have an exclusive effect on cancer cells; they impact the whole body. Some men with prostate cancer have told their physicians they’d rather die than take antiandrogens, which are sometimes referred to as “medical castration.” And women on estrogen modulators report symptoms of menopause, from hot flashes to depression, that disrupt their lives daily.
Pamela Munster, MD, hopes the implants will dial down the side effects of these drugs. She directs early-phase clinical trials and co-leads the Center for BRCA Research at UCSF’s Helen Diller Family Comprehensive Cancer Center, and her lab created the implants. She says they could be relatively simple to put in. Munster compares the concept and materials to the intrauterine device, a contraceptive that women replace about every five years.
“But they’re a lot, lot smaller – see how tiny?” Munster says, displaying a scan of the silicone devices embedded in a prostate. Inserted with a biopsy needle, they’re just a few millimeters long. Munster envisions a future in which BRCA-positive patients could safely choose the implants over mastectomies, having them removed temporarily if they opt to give birth and breastfeed.
“Whenever I see a patient with a BRCA mutation who is 25, and we talk about planning a risk-reducing mastectomy, that individual will ask me, ‘Is there nothing else?’” says Munster, who carries a BRCA2 mutation herself. “Localized drug delivery is so new, and it is very difficult to prove that something can prevent cancer. It takes a long view. It takes precise engineering. But patients need this. My own children might benefit from this one day.”
DNA-Driven Solutions
Targeting Tumors’ Genetic Fuel
There are many kinds of cancer, but they all have one theme in common: They’ve broken free of the rules that govern cell growth, repair, and death. Genetic research aims to figure out why the cancer causes so much trouble. Many mutations can drive it, hitting the gas pedal on cellular growth or cutting the brakes that ought to slow it down.
This knowledge has led to new therapies that target specific genetic mutations by throwing a wrench into the cancer’s replication machinery. But some mutations have been difficult to disrupt. For many years, researchers considered KRAS, the most common genetic driver of tumors, to be the undruggable “Death Star” of cancer.
The KRAS gene makes a protein that guides cellular growth. “KRAS is normally doing good things in cells,” explains Charles Craik, PhD, a professor of pharmaceutical chemistry at UCSF. “But when it mutates, then the brakes are off, and it’s a runaway train.”
Kevan Shokat, PhD, a UCSF biochemist and Howard Hughes Medical Institute investigator, succeeded where others had given up. He collaborated with Jonathan Ostrem, MD, PhD, a graduate student then and assistant professor of hematology and oncology now, and Ulf Peters, PhD, a postdoctoral fellow at the time. Together, they searched for new ways to manipulate KRAS activity and understand the structure of the protein it makes – ultimately revealing a previously unknown pocket on the surface of mutated KRAS protein to which drugs can bind.
It was a big deal. Shokat received the Sjöberg Prize and the National Academy of Sciences’ Award for Scientific Discovery. The research inspired a flurry of drug development, and in 2021, sotorasib became the first therapy approved by the U.S. Food and Drug Administration (FDA) for a cancer caused by KRAS mutations. Craik says this lung cancer drug could become just one of many new regimens tailored to KRAS-fueled tumors.
“The mutant KRAS, the bad actor, is only in the tumor cells,” Craik says. “This target is much more specific than just rapidly dividing cells. When you look at how chemotherapies work, you’re just poisoning the cancer cells more than you’re poisoning the normal cells. Now we have a chance to do true precision medicine.”
Exciting, right? Except cancer is tricky. As thrilling as sotorasib’s launch was, Craik and Shokat anticipated that some KRAS-driven lung tumors would prove resistant to the drug. It doesn’t happen to every patient, but it happens. The cancer adapts, and the treatment stops working. It’s called acquired resistance.
“Sure enough, some of those patients got resistance,” Craik says. “The tumor was shrinking, but all of a sudden, it starts coming back.”
There’s also inherent resistance: additional genetic tweaks or chemical pathways that enable a patient’s particular cancer to bypass a treatment. Craik adds: “While KRAS is present in lung, colon, and pancreatic cancers, sotorasib currently only works in lung cancer. It doesn’t work in colon cancer. It’s not that you develop resistance in that case. It doesn’t work right from the get-go.”
That doesn’t mean hope is lost. Researchers are investigating treatments that deliver a one-two punch: first disrupting the mutated KRAS, then disabling the cancer’s other resistance tools. Craik and Shokat have developed a new therapy that pulls mutated KRAS to the surface of cells, essentially flagging them as cancer. Then an immunotherapy can help the body remove all the flagged cells. They think this approach might work especially well combined with sotorasib.
“If we can help the immune system spot tumors it wasn’t finding before, maybe we can add durability to these targeted drugs,” Craik says. He adds that the research is ongoing – not quite ready for a clinical trial, but full of potential: “If we’re successful, we’re not limited. We could do this with other irreversible inhibitors against other genetic targets. This isn’t just a KRAS story.”
A Well-Armed Resistance
Reprogramming Immune Cells to Attack Cancer
Theoretically, your immune system should hunt down cancer cells and eliminate them before they can turn into tumors. The immune system is kind of like a security team, monitoring the body and removing sources of bad behavior. Your T cells are essential members of this team.
Unfortunately, cancer has clever ways to dodge security. It can secrete molecules or recruit other cells that stop T cells from doing their job, sort of like slipping these immune guards a strong sedative. It can also cloak itself, changing how it expresses antigens. Cells usually display antigens on their surface like an identity card, and the T cells check whether they belong in your body or need to get booted out. By changing how they express antigens, cancer cells basically become invisible – free to slip past the T-cell bouncers and cause some Road House-level mayhem.
Immunotherapies treat cancer by overcoming these defenses. A relatively new approach, chimeric antigen receptor T-cell (CAR T) therapy, has quickly become one of the most promising tools in the treatment arsenal.
“The T cells can be pretty much blind to the tumor,” explains Justin Eyquem, PhD, an assistant professor of microbiology and immunology. “One way to make the T cells see the tumor again is to add a new gene to the T cells.”
In CAR T, scientists take some of a patient’s T cells and genetically engineer them, training the T cells to recognize a different antigen on the cancer and latch onto it. Then the medical team injects the revamped T cells back into the patient, where they can track down the cancer cells much more easily than before. So far, CAR T works well for certain types of blood cancers, like leukemia.
“These leukemia patients had already tried all the other treatments available,” Eyquem says. “Remarkably, about 50% to 90% of them get what we call a complete response from the CAR T. That means a few weeks after one injection of these T cells, you cannot detect the cancer in their body anymore. That is a paradigm shift.”
The CAR T targets an antigen that’s expressed exclusively by the leukemia and by B cells, a kind of white blood cell. You can’t live long with zero B cells because your body needs them to make antibodies, which fight off infection. But people receiving CAR T can survive by getting infusions of antibodies instead.
It’s not all amazing news, though. For some patients receiving CAR T, the cancer eventually returns. Perhaps the patient’s cancer evolves, cloaking its antigens even better than before. Maybe the modified CAR T cells just don’t persist long enough to attack all the cancer. Plus, blood cancers aren’t quite like solid cancers. The latter involve tumors, which have more immune defenses – including dense networks of blood vessels that feed and protect them.
Eyquem and other researchers are working to improve CAR T, making it more effective and expanding its reach beyond blood cancers. For example, his team has invented a new, ultrasensitive type of receptor that could supercharge T cells, helping them spot even the lowest levels of cancer antigens. Another UCSF team, led by Julia Carnevale, MD, an assistant professor of hematology and oncology, recently used CRISPR technology to identify genes that can boost engineered T cells. So far, in mice with tumors, these tweaked T cells last longer and work more effectively than non-boosted T cells.
Meanwhile, other UCSF scientists have created combination receptors for CAR T, which require several antigens to activate the T cells. You know how two-factor authentication makes you log in and enter a code from your phone to confirm it’s you? Combination receptors work kind of like that. They could be useful for cancers with antigens that are also expressed in healthy organs. You want the T cells trained to attack the cancer – only the cancer, not your perfectly serviceable brain cells.
To accelerate its production of CAR T cells, UCSF recently started manufacturing them at a new facility run by Thermo Fisher Scientific. Researchers are also trying to find affordable ways to scale up treatments like CAR T. Right now, it’s extremely expensive to produce made-to-order T cells for each patient.
“One day, we could move from cell therapy to gene therapy,” Eyquem says. “The idea is not to take your T cells out and engineer them but instead inject the vector that is going to engineer the cells directly into the patient. It’s still a bit science fiction, but it’s exciting.”
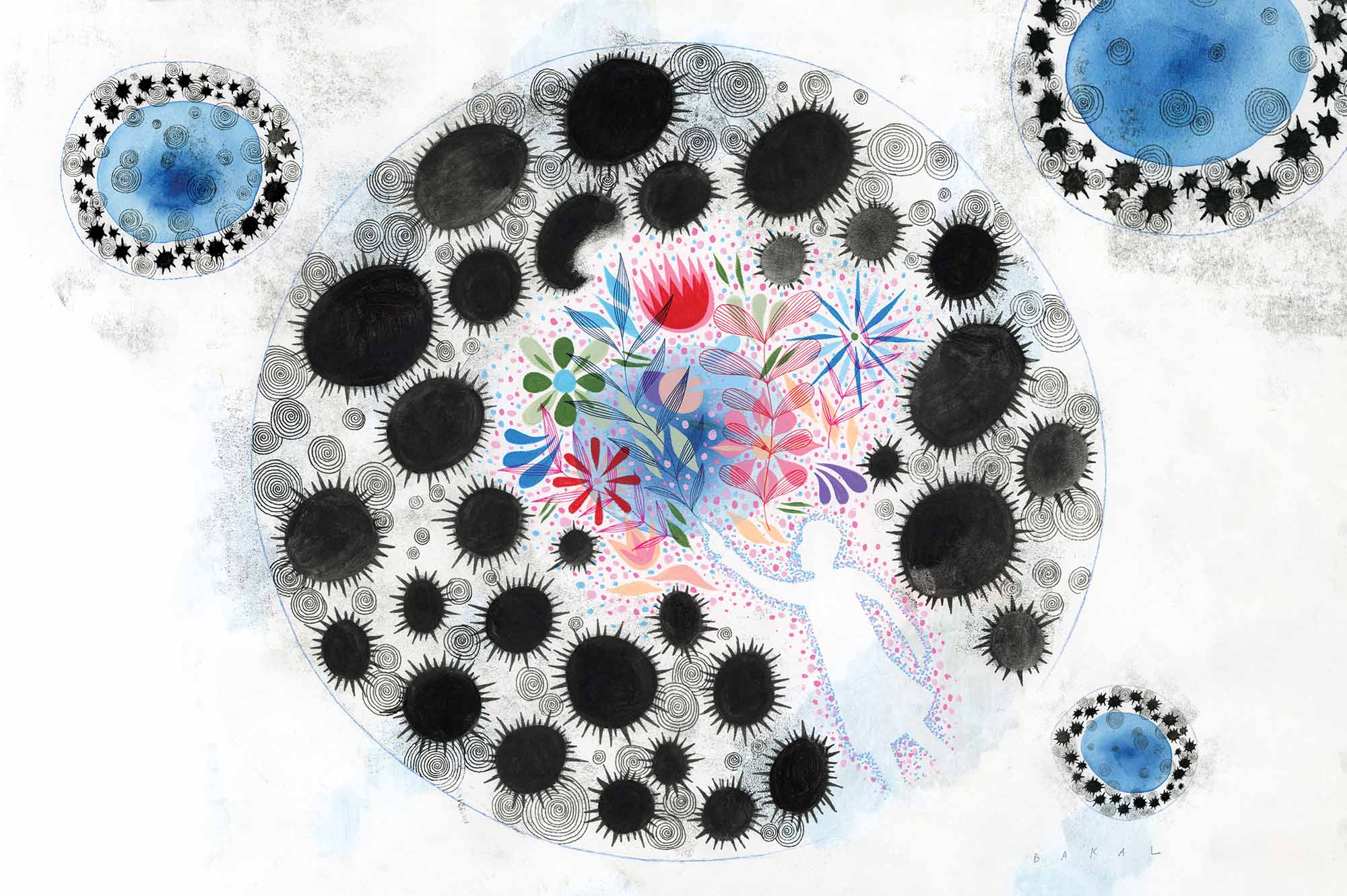
High-Tech Blood Tests
Catching Cancer When It’s Easier to Eliminate
Lacking cures for late-stage cancers, some researchers have focused on helping people get diagnosed long before their tumors turn deadly. Early-detection tools for some tumors already exist: colonoscopies, mammograms, blood tests for a worrisome prostate biomarker. But many kinds of cancer have no such test. By the time they're discovered, damage is already done.
“For the deadliest, most common form of ovarian cancer, we’re still diagnosing at stage 3 and 4 about 90% of the time,” says Jocelyn Chapman, MD, the Berson Family Professor of Gynecologic Cancer. “I’d like to detect ovarian cancer when it’s at stage 0, when we can do surgery and that’s the end of that.”
Chapman and her colleagues are trying to create a blood test for ovarian cancer. They’re part of a new frontier in early detection, as biotech companies race to develop “liquid biopsies” that could curb cancer deaths.
Scientists have known for a while that cancer cells release genetic material into the bloodstream. The trick to an ideal blood test lies in figuring out which parts of that material reliably predict cancer, whether it’s one type or many. The test needs to be sensitive enough to pick up the cancer signal. But it also needs to be specific enough to avoid alarming thousands of healthy patients every year. Each false-positive result could kick off a series of expensive, unnecessary procedures – not to mention the emotional whiplash of hunting for tumors that you don’t actually have.
To solve this problem, Chapman is collaborating with Nadav Ahituv, PhD, a UCSF professor of bioengineering and therapeutic sciences and director of the Institute for Human Genetics. His research focuses on neomers: short segments of DNA that are altered in cancer, but not in normal tissue.
“Neomers are scattered all over the genome, which makes them a bit more useful than just looking at one or two gene mutations,” Chapman says. “It’s not like we can look for a single strand of DNA that changes in one way and then go, ‘Ah-ha, that’s ovarian cancer!’ Instead, we’re looking for a specific kind of pattern. When you see that pattern over and over again, it’s like a cancer fingerprint.”
If researchers like Chapman and Ahituv succeed, we might one day look back on the era when cancer spread silently in the body and call it archaic – not unlike the time before fire alarms, when people were usually choking on smoke before anyone had a clue the house was burning.
“At the beginning of my career, I thought all I was going to do was operate on people, give them chemotherapy, and watch them die a few years later,” Chapman says. “I would have given those patients some time. But the technology has dramatically improved. I’m hopeful that this will actually change before I retire. Maybe we can give people not just a few years, but many, many years.”
Nowhere to Hide
Lighting Up Cancer Wherever It Lives
For many years, the best way to tell if a tumor had metastasized was by using anatomic imaging: scans that combine a series of X-ray images into a rough picture of your insides.
But anatomic imaging is not a precise way to track down all your cancer cells. Thomas Hope, MD, a professor of radiology, led a clinical trial that helped UCSF and UCLA gain FDA approval to provide the first PSMA PET scans for U.S. prostate cancer patients. Since then, the new imaging technique has taken off. Today, patients across the country are getting the scans.
PSMA stands for prostate-specific membrane antigen, a protein found on most prostate cancer cells. The new technique uses positron emission tomography (PET) plus a drug that binds to PSMA, illuminating prostate cancer cells anywhere they reside in the body.
“Before PSMA PET, we didn’t know where the disease was,” Hope says. “In essence, PSMA PET shines a light on it.”
Once doctors know exactly where the cancer is, they can get to work removing it. Peter Carroll, MD, MPH, UCSF’s Derr-Chevron Distinguished Professor of Prostate Cancer and Taube Family Distinguished Professor of Urology, and Hao Nguyen, MD, PhD, the Grinold Professor of Urology, are bringing PSMA-targeted imaging into the operating room. Participants in their clinical trial get a compound that attaches to PSMA. Then surgeons use a camera that highlights those cells, helping them remove traces of cancer that might have been impossible to spot otherwise, even with the latest robotic surgical tools.
“The cure rate for patients with high-risk prostate cancer has not changed over the last 20 years,” Nguyen says. “About 30% still get a recurrence after surgery. We want to find all the invisible bits of cancer and remove them completely because that could save lives and reduce the need for additional treatment.”
Meanwhile, Hope is collaborating with researchers like Vadim Koshkin, MD, an assistant professor of hematology and oncology, to investigate ways of using PSMA-targeted technology to treat advanced cancers that can’t be eliminated with surgery alone. The treatments work like a key that only fits a PSMA-specific lock, delivering damage to cancer cells and skipping healthy ones. They just launched a trial for prostate cancer patients using radiation therapy that binds to PSMA, plus a drug that increases PSMA expression. In theory, the combination could outperform existing treatments, possibly with fewer side effects.
The improved imaging made possible by PSMA PET will inspire much more research that aims to customize cancer treatments, Hope says. “Before, we gave systemic therapy because we didn’t know that, for example, a patient had disease in only one lymph node. Now that we know exactly where the cancer is, maybe we get to do something different – something better.”